If you find Complexity Thoughts interesting, follow us! Click on the Like button, leave a comment, repost on Substack or share this post. It is the only feedback I can have for this free service. The frequency and quality of this newsletter relies on social interactions. Thank you!
→ Don’t miss the podcast version of this post: click on “Our Podcast” above!
Very busy in the last couple of weeks with a bunch of important events (among which, the first NetworksDays!) and pressing administrative work (I will never stop claiming that, especially at the Italian universities, we should do less admin stuff and be allowed to do what we do better: SCIENCE!). Therefore, in this Issue I will cover less papers than usual. Hopefully I’ll get back the super-powers from the next week.
From the lab
Topological conditions drive stability in meta-ecosystems
On a global level, ecological communities are being perturbed at an unprecedented rate by human activities and environmental instabilities. Yet, we understand little about what factors facilitate or impede long-term persistence of these communities. While observational studies indicate that increased biodiversity must, somehow, be driving stability, theoretical studies have argued the exact opposite viewpoint instead. This encouraged many researchers to participate in the ongoing diversity-stability debate. Within this context, however, there has been a severe lack of studies that consider spatial features explicitly, even though nearly all habitats are spatially embedded. To this end, we study here the linear stability of meta-ecosystems on networks that describe how discrete patches are connected by dispersal between them. By combining results from random-matrix theory and network theory, we are able to show that there are three distinct features that underlie stability: edge density, tendency to triadic closure, and isolation or fragmentation. Our results appear to further indicate that network sparsity does not necessarily reduce stability, and that connections between patches are just as important, if not more so, to consider when studying the stability of large ecological systems.
Fundamental constraints to the logic of living systems
I have already described this paper in a previous dedicated post:
I have learned so much from collaborating with such a dream team of authors. This paper is a little gem with a lot of food for #ComplexityThoughts.
It has been argued that the historical nature of evolution makes it a highly path-dependent process. Under this view, the outcome of evolutionary dynamics could have resulted in organisms with different forms and functions. At the same time, there is ample evidence that convergence and constraints strongly limit the domain of the potential design principles that evolution can achieve. Are these limitations relevant in shaping the fabric of the possible? Here, we argue that fundamental constraints are associated with the logic of living matter. We illustrate this idea by considering the thermodynamic properties of living systems, the linear nature of molecular information, the cellular nature of the building blocks of life, multicellularity and development, the threshold nature of computations in cognitive systems and the discrete nature of the architecture of ecosystems. In all these examples, we present available evidence and suggest potential avenues towards a well-defined theoretical formulation.
Foundations of network science and complex systems
What Emergence Can Possibly Mean
I hope to have the time to write soon a dedicated post to emergence. In the meanwhile:
We consider emergence from the perspective of dynamics: states of a system evolving with time. We focus on the role of a decomposition of wholes into parts, and attempt to characterize relationships between levels without reference to whether higher-level properties are “novel” or “unexpected.” We offer a classification of different varieties of emergence, with and without new ontological elements at higher levels.
See also previous posts:
This is an interesting paper that adds important advances on the current debate about assembly theory. We have covered the debate in the past:
We formally prove the equivalence between Assembly Theory (AT) and Shannon Entropy via a method based upon the principles of statistical compression that belongs to the LZ family of popular compression algorithms. Such popular lossless compression algorithms behind file formats such as ZIP and PNG have been shown to empirically reproduce the results that AT considers its cornerstone. The same results have also been reported before AT in successful application of other complexity measures in the areas covered by AT such as separating organic from non-organic molecules and in the context of the study of selection and evolution. We demonstrate that the assembly index is equivalent to the size of a minimal context-free grammar. The statistical compressibility of such a method is bounded by Shannon Entropy and other equivalent traditional LZ compression schemes, such as LZ77 and LZW. We also demonstrate that AT, and the algorithms supporting its pathway complexity, assembly index, and assembly number, define compression schemes and methods that are subsumed into algorithmic information theory. We conclude that the assembly index and the assembly number do not lead to an explanation or quantification of biases in generative (physical or biological) processes, including those brought about by (abiotic or biotic) selection and evolution, that could not have been arrived at using Shannon Entropy, or that have not been already reported before using classical information theory or algorithmic complexity.
Evolution
The overlooked biodiversity loss
A holobiont describes an organism together with the diverse community of microorganisms (such as bacteria, fungi, viruses, and other microbes) living in or on it. It is a collective (composed of the host organism and its microbial symbionts) that functions as a single ecological unit. From this point of view, the host and its microbes interact so closely that they can be considered a single entity in ecological/evolutionary, adapting and evolving together in response to environmental pressures.
A good example is given by the human body with its gut microbiome (the community of microbes in the digestive system), regulating from digestion to immune function and jointly contributing to health and adaptation. A great example of two systems that form a more complex and integrated system than the host alone.
As most life-forms exist as holobionts, reduction of host-level biodiversity drives parallel habitat losses to their host-adapted microorganisms. The holobiont concept helps us to understand how species are habitats for – often ignored – coevolved microorganisms also worthy of conservation. Indeed, loss of host-associated microbial biodiversity may accelerate the extinction risks of their host.
Ecosystems
Ecosystem synchrony: an emerging property to elucidate ecosystem responses to global change
Global change induces multiple perturbations that can directly and indirectly affect the dynamic of ecosystems. To date, studies have primarily focused on ecological responses at lower levels of biological organization using population and community synchrony. Ecosystem synchrony, that is, the similarity in the temporal fluctuations of an ecosystem function between multiple ecosystems, is an emergent property of ecosystems that is affected by the interplay between biotic and abiotic dynamics. Ecosystem synchrony represents an integrative approach to quantify the spatial and temporal extents of the coordinated ecosystem responses to natural and human-induced perturbations. It opens avenues for advances in studies on ecological dynamics which could ultimately deliver future advanced warning methods and actionable management.
Understanding ecosystem responses to global change have long challenged scientists due to notoriously complex properties arising from the interplay between biological and environmental factors. We propose the concept of ecosystem synchrony – that is, similarity in the temporal fluctuations of an ecosystem function between multiple ecosystems – to overcome this challenge. Ecosystem synchrony can manifest due to spatially correlated environmental fluctuations (Moran effect), exchange of energy, nutrients, and organic matter and similarity in biotic characteristics across ecosystems. By taking advantage of long-term surveys, remote sensing and the increased use of high-frequency sensors to assess ecosystem functions, ecosystem synchrony can foster our understanding of the coordinated ecosystem responses at unexplored spatiotemporal scales, identify emerging portfolio effects among ecosystems, and deliver signals of ecosystem perturbations.
A general consumer-resource population model
Food-web dynamics arise from predator-prey, parasite-host, and herbivore-plant interactions. Models for such interactions include up to three consumer activity states (questing, attacking, consuming) and up to four resource response states (susceptible, exposed, ingested, resistant). Articulating these states into a general model allows for dissecting, comparing, and deriving consumer-resource models. We specify this general model for 11 generic consumer strategies that group mathematically into predators, parasites, and micropredators and then derive conditions for consumer success, including a universal saturating functional response. We further show how to use this framework to create simple models with a common mathematical lineage and transparent assumptions. Underlying assumptions, missing elements, and composite parameters are revealed when classic consumer-resource models are derived from the general model.
Neuroscience and Cognitive Sciences
Intrinsic cortical activity forms traveling waves that modulate sensory-evoked responses and perceptual sensitivity. These intrinsic traveling waves (iTWs) may arise from the coordination of synaptic activity through long-range feature-dependent horizontal connectivity within cortical areas. In a spiking network model that incorporates feature-selective patchy connections, we observe iTW motifs that result from shifts in excitatory/inhibitory balance as action potentials traverse these patchy connections. To test whether feature-selective motifs occur in vivo, we examined data recorded in the middle temporal visual area (Area MT) of marmosets performing a visual detection task. We find that some iTWs form motifs that are feature selective, exhibiting direction-selective modulations in spiking activity. Further, motifs modulate the gain of target-evoked responses and perceptual sensitivity if the target matches the preference of the motif. These results suggest that iTWs are shaped by the patchy horizontal fiber projections in the cortex and can regulate neural and perceptual sensitivity in a feature-selective manner.
Human behavior
Analogies for modeling belief dynamics
Belief dynamics is at the core of many challenges that our societies currently face, from misinformation to polarization and violent conflict. They emerge from the interaction between cognitions and social networks over time.
Belief dynamics has an important role in shaping our responses to natural and societal phenomena, ranging from climate change and pandemics to immigration and conflicts. Researchers often base their models of belief dynamics on analogies to other systems and processes, such as epidemics or ferromagnetism. Similar to other analogies, analogies for belief dynamics can help scientists notice and study properties of belief systems that they would not have noticed otherwise (conceptual mileage). However, forgetting the origins of an analogy may lead to some less appropriate inferences about belief dynamics (conceptual baggage). Here, we review various analogies for modeling belief dynamics, discuss their mileage and baggage, and offer recommendations for using analogies in model development.
Biological computing
Biomolecular computing systems: principles, progress and potential
This review paper is immense. I should be ordering my ideas and write a dedicated post to this too, at some point. Highlights:
The notion of computation is none other than a systematic way of processing information, and thus computation is central to the function of biological systems, as it is crucial for complex man-made machinery.
Whereas biological computing is ubiquitous in living systems, the capacity to engineer new biological computing systems will open the way to an unprecedented level of rational control over living matter that can be used in all areas of biological engineering and medicine
Current engineering effort is split between biochemical systems that function in carefully constituted settings and biological systems that operate in living cells or cell ensembles. The two approaches are complementary because biochemical systems show what is possible in principle, whereas biological systems must deal with the complexity of the host and thus are at this point simpler and smaller in scale.
The construction of molecular computing systems has been inspired by known theoretical models of computation, such as state machines and logic and analogue circuits. Each model is best suited for a different class of tasks.
The logic circuits model has spawned a large number of implementations both in the test tube and in living cells, with the basic building blocks comprising DNA oligomers in the test tube and re-engineered regulatory switches in living cells. Recent achievements include neural-like network with associative memory made of DNA switches, a trainable ribozyme-based molecular network, a number of distributed logic gates in bacteria and yeast and a cell-type classifier for cancer cell detection and destruction.
Molecular systems inspired by state machines were implemented with both biochemical and biological approaches, resulting in molecular finite automaton and recombinase-based counter.
The task of information processing, or computation, can be performed by natural and man-made 'devices'. Man-made computers are made from silicon chips, whereas natural 'computers', such as the brain, use cells and molecules. Computation also occurs on a much smaller scale in regulatory and signalling pathways in individual cells and even within single biomolecules. Indeed, much of what we recognize as life results from the remarkable capacity of biological building blocks to compute in highly sophisticated ways. Rational design and engineering of biological computing systems can greatly enhance our ability to study and to control biological systems. Potential applications include tissue engineering and regeneration and medical treatments. This Review introduces key concepts and discusses recent progress that has been made in biomolecular computing.
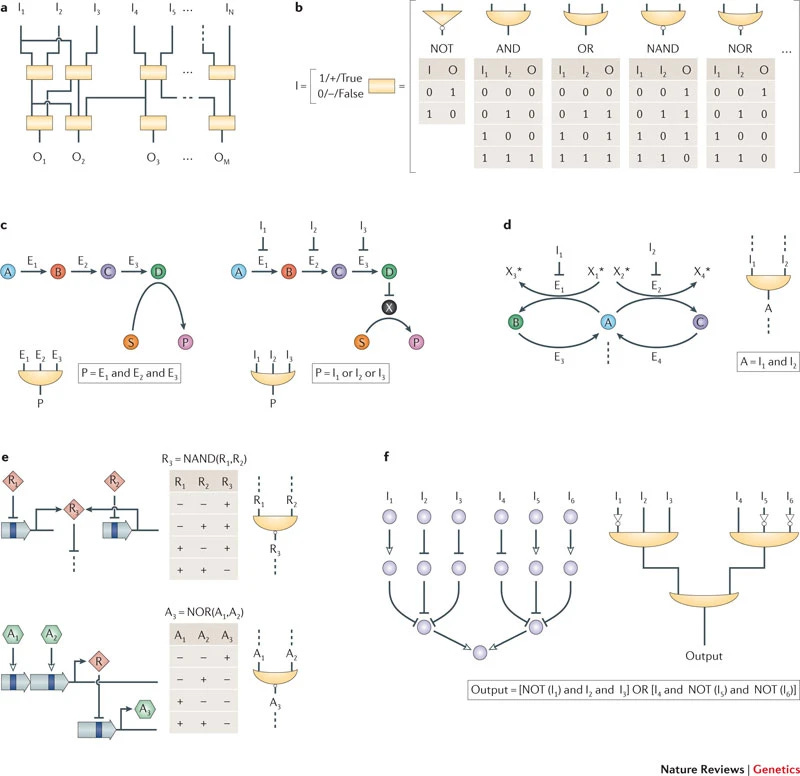
I am a researcher who wrote almost 95 papers on academia.edu about Complexity and Complexification related issues.